Powder Comparison: Standards for DED Material Substitutes
What powder properties lead to performance similar to a DED powder? Basic flowability energy, particle density and particle morphology are key candidates.
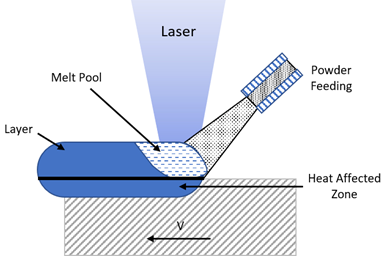
In DED, a laser beam melts powder as it deposits, metallurgically fusing it with a thin surface layer of substrate. This AM method has a low material usage rate, excellent for applications like aerospace. Images courtesy of Freeman Technology.
Editor’s note: This piece is based on research conducted by Jamie Clayton of Freeman Technology, Lisa Markusson, previously of GKN Aerospace Sweden AB and Lars Östergren currently of GKN Aerospace Sweden AB.
Directed energy deposition (DED), also called laser metal deposition (LMD), uses lasers to fuse materials as they are being deposited. This mid-process fusion differentiates DED from binder jetting and powder bed fusion while also making the technique suitable for repair work. DED causes lower thermal stress than arc welding or thermal spraying, and the ease of switching between materials when using this technique improves its versatility.
Optimizing DED relies on selecting powders that will process well and produce components and repairs with desirable properties. Many of these powders are developed specifically for DED — so what can users do when supply chain demands leave them with shortages of powder?
Researchers at Luleå University of Technology and GKN Aerospace Engine Systems set out to discover which particle and powder properties correlate to similar DED performance and build qualities. They compared five Inconel 718 powder samples — one powder which GKN regularly uses (powder C), as well as four others (powders A, B, D and E) — from four different suppliers.
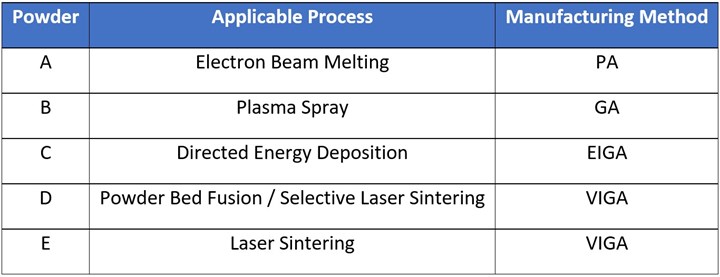
The powders used in this experiment were sold for various processes, and their manufacturing methods resulted in different particle morphologies. The manufacturing methods were plasma atomization (PA), gas atomization (GA), vacuum inert gas atomization (VIGA) and electrode induction gas atomization (EIGA).
The Experiment
To assess the print performance and deposit quality of each powder, the researchers printed single and multi-bead deposits onto Inconel 718 plates. Process parameters were optimized for powder C and left the same for each powder. Measurements of the powder supplied versus the weight of the deposit determined powder efficiency; while defects, geometry, and microstructure determined deposit quality.
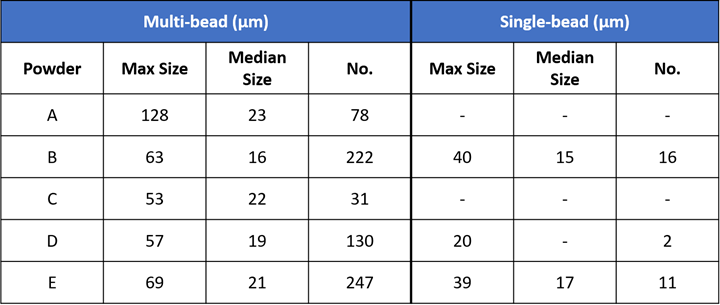
Powders A and C create slightly larger pores than the other powders, but the number of pores they create is much smaller.
While all four alternative powder supplies offered higher efficiency than powder C, the higher variability of powder E made it far less desirable. In respect to bead quality, pore number and pore size, powder A produced results closest to powder C. From here, the researchers compared several physical characteristics to determine which properties could differentiate powders A and C from the others.
Before beginning research, GKN’s specifications for DED powders referenced composition, manufacturing method, Hall Flow Index and particle size distribution. Over the course of the study, however, particle size and manufacturing method proved unable to successfully predict powder A as the closest performance match to powder C, and while Hall Flow Rate suggested the two were similar, all five powders still passed GKN’s established specification on the parameter.
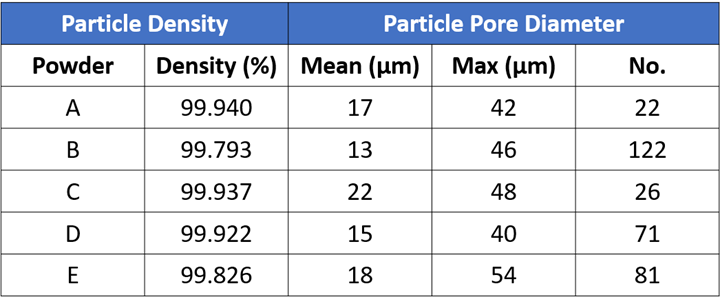
As with the data on the deposits, the density and pore data for the powder particles suggests similarities between powders A and C. The two are closest in density, and have similarly smaller numbers of pores.
Porosity in the feedstock powder appeared to be better linked to porosity in the deposit, and powders A and C were the closest analogues in specific energy (which quantifies unconfined flow behavior). Similarities in the difference between the powders’ conditioned bulk density and bulk density after 50 taps of vibrational consolidation also distinguished powders A and C from their counterparts.
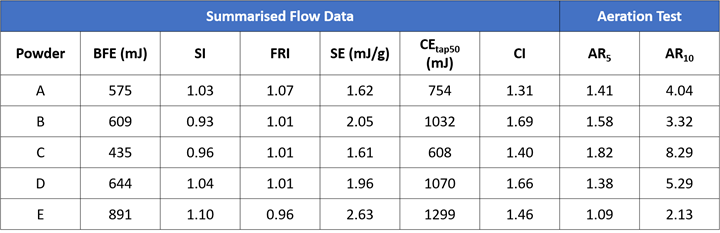
Dynamic flow properties are most similar between powders A and C, and provide a high degree of differentiation.
Conclusions
Ultimately, the researchers concluded that powder efficiency was correlated with basic flowability energy and deposit pore frequency was correlated with particle pore frequency. While they acknowledge the limited data set, they also argue that a small selection of highly similar powders is representative of the practical challenge DED users face when assessing alternative supplies.
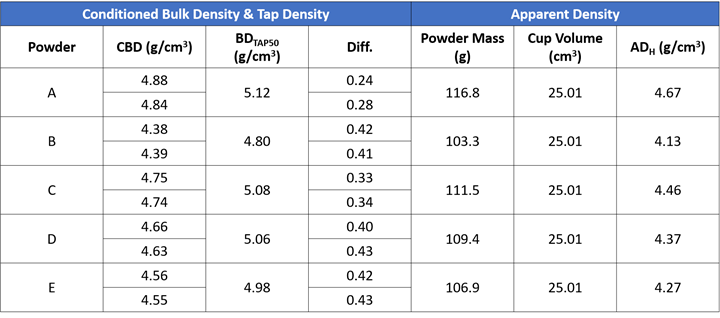
Particle density data reveals powders A and C as the densest particles out of the selection, with the bulk density after consolidational tapping (BD50Taps) suggesting the two also have the highest packing efficiency.
Therefore, they say most users can learn from four primary takeaways. First, dynamic testing provides far greater insight into flowability behavior and provides more robust differentiation between samples than the Hall Flow Index. Second, flowability directly impacts performance metrics such as powder efficiency. Third, part quality — especially bead porosity — is highly dependent on the density and porosity of powder particles. Lastly, particle shape influences powder suitability.
For a new metal powder specification, the researchers say any new metal powder specifications should include notes on basic flowability energy, along with a descriptor of particle morphology and particle density.
About the Study Authors
Jamie Clayton is the operations director at powder characterization company Freeman Technology. Based at the company’s headquarters in Tewkesbury, UK, he is responsible for overall management of company activities, including the R&D, production sales and customer support teams.
Lisa Markusson previously worked as a graduate engineer at Luleå University of Technology and then as a production manager at GKN Aerospace Sweden AB. She is currently project coordinator for e-mobility at AFRY in Stockholm, Sweden.
Lars Östergren is a design engineer for concept development in additive manufacturing at GKN Aerospace Sweden AB, also based in Stockholm.
Related Content
Can the U.S. Become Self-Sufficient in Aerospace Alloy Metal Powders?
6K’s technology can upcycle titanium and nickel-alloy parts into additive manufacturing powder. Here is how the circular economy helps national security.
Read MoreWhat Is Neighborhood 91?
With its first building completely occupied, the N91 campus is on its way to becoming an end-to-end ecosystem for production additive manufacturing. Updates from the Pittsburgh initiative.
Read MoreDo Distributors Dream of Digital Inventory? Würth Additive Group Does
It’s more than a dream for Würth Additive Group and its parent company, in fact. Along with supplying additive equipment, the group is now developing solutions for sourcing 3D printed parts in a reliable, elastic digital inventory model.
Read More3D Printed Golf Putter Shafts: AM Tailors Weight Distribution for an Optimized Putting Stroke
Snarr3D offers high-performance golf putters with aesthetic features made possible through 3D printing. In manipulating the weight distribution of the golf putter shaft, Snarr3D aims to enhance accuracy and performance to give golfers the equipment suited to their individual game.
Read MoreRead Next
Robots Combine for Faster DED Build Rate (Video)
The Medusa system merges multiple robots into a single system for efficient additive manufacturing of large metal parts.
Read MoreHow One Device Inspects Both Parts and Powder in Metal AM
A scanning electron microscope analyzes the shape and composition of grains of powder as well as inclusions and voids in the part’s surface.
Read MoreOptimizing Metal Powders for Additive Manufacturing
What makes a good metal powder for additive manufacturing? Case study data highlights the value of particle size and shape, powder flowability, and bulk density.
Read More