Is a Gel Bath the Key to Scalable, Affordable 3D Printed Ceramics?
3D printed polymer-derived ceramics (PDCs) could find wider application through a process developed at the University of Texas at Dallas that extrudes preceramic material in a self-healing bath for support through printing and curing.
Share

Could extrusion 3D printing coupled with an innovative support strategy make it easier and more affordable to produce high-performance, complex ceramic parts? Photo Credits: University of Texas at Dallas
Ceramics present a compelling use case for additive manufacturing (AM). The materials are difficult to machine and challenging to cast. But they offer great potential in terms of thermal properties, lightweighting and corrosion resistance. Could 3D printing be a solution to achieving cost-effective, large, geometrically complex and high-performance parts with these materials?
From the perspective of Dr. Mijad Minary at the University of Texas at Dallas (UTD), yes and no. No, because stereolithography and powder bed fusion come with size limitations and are inhibited by ceramics’ low laser absorption. And possibly no because methods such as binder jetting require the introduction of additives which can inhibit final part density or reduce durability in high-temperature applications. And perhaps no because all of the above techniques print layer-by-layer, which reduces Z strength and potentially introduces interlayer stress concentration. But also yes, because polymer-derived ceramics (PDCs) are opening another path to 3D printable ceramics, one that can achieve stronger, larger parts produced at lower cost and from purer material.
Dr. Minary’s lab at UTD has developed a process for manufacturing ceramic parts by extruding and postprocessing preceramic polymer, the precursor to PDC. Compared to 3D printing with powder-based ceramic materials, “This method is advantageous because we know a lot more about polymers and their processing, and we have more flexibility to shape them in terms of additive manufacturing,” he says. Robocasting (or robotic material extrusion) 3D printing combined with an unusual support technique has resulted in a scalable, low-cost method for manufacturing high-performance ceramic parts.
3D Printing with Polymer-Derived Ceramics
As the name implies, PDCs involve polymers that can be transformed into a ceramic. This class of materials is often liquid at room temperature, but can be cured into solid, crosslinked polymer and pyrolyzed into ceramic through the application of heat. Once fired, the material exhibits high-temperature and corrosion resistance suitable for applications in defense, energy and microelectromechanical systems. Similar to thermoset plastics, PDCs cannot be remelted or converted back into resin once they’ve made this transformation. These characteristics combined with the low viscosity of the material at room temperature (somewhere between the consistency of motor oil and honey) mean that it is not possible to extrude the material like a standard fused filament fabrication (FFF) filament or robocasting paste.
“Unless the polymer is crosslinked, it cannot hold its shape,” Minary explains. “But, once it is crosslinked, you cannot extrude it; it just blocks your nozzle.” The material, therefore, must be printed in its preceramic resin state, before being crosslinked and pyrolyzed.
The specific polymer-derived ceramic used in the development of UTD’s process is silicon oxycarbide (SiOC). This PDC requires a steady temperature of 160°C for two hours to cure into a crosslinked polymer, and around 900°C for several hours for pyrolyzation. Initially, Minary’s lab focused on modifying the robocasting extrusion process to trigger the crosslinking reaction, but heating the substrate to cure the deposited material was effective only for the first few layers of a print, while applying heat at the nozzle did not provide a sufficient exposure time. Additives mixed with the SiOC could have produced a 3D printable paste, but the team wanted to maintain the ceramic’s purity as much as possible.
Ultimately, the method that made it possible to 3D print with preceramic SiOC was not to extrude it in open air, but instead into a thixotropic support bath. The bath used is a gel-like solution of mineral oil and silica nanoparticles that is solid enough to support the preceramic polymer yet liquid enough to enable the nozzle to move through it without leaving a trail in its wake.

This airfoil and two helical springs are still in their preceramic state, supported and held in place by the thixotropic bath. This technique enables complex 3D printed ceramics without adding support structures.
Minary describes the bath’s consistency as similar to another shearing liquid that might be found in the nearest refrigerator. “Ketchup holds itself like a solid and doesn’t flow, but it can be sheared with a knife or by shaking the bottle,” he explains. “It goes through a solid-fluid-solid transition.”
The mineral oil-silica nanoparticle bath works in the same way, transforming from solid to fluid in a sheared area two to three times the diameter of the print nozzle to allow for the deposition of the preceramic polymer, and then “heals” around the print and resumes its solid behavior when the nozzle moves on. The sheared area extends slightly below the plane of the nozzle tip, clearing a path for the preceramic polymer to be deposited right on top of, and to merge with, any existing layer. As an additional benefit, the bath is transparent, which enables operators to watch and monitor the build in situ. See the printer in action in the video below:
Thixotropic Support Bath as a 3D Printing Parameter
Interfacial tension between the extruded material and the bath makes this 3D printing technique possible, while the yield stress of the bath is what limits the process, Minary says. Once researchers achieved the correct bath viscosity and rheology by adjusting the amount of silica, they still had to tune the speed of the printhead to ensure the desired shearing effect. Moving the nozzle too slowly might not produce enough of a fluid region, while moving it too quickly could introduce recirculation, causing the preceramic polymer to mix in with the gel. Tests for this research were ultimately 3D printed at a speed of about 50 mm/second. “You can move the nozzle faster, but you have to work with the rheology of the bath to ensure that the fluid region is not too big,” Minary says.
This 3D printing method offers all the geometric freedom of a thermoplastic FFF or robocasting 3D printer, plus more. No support structures are necessary, which saves material and simplifies both printing and postprocessing. And while this technique can be applied layer-by-layer, it also opens new pathing options not typically available through extrusion-style 3D printers such as omnidirectional printing, illustrated via the spiral coil below.
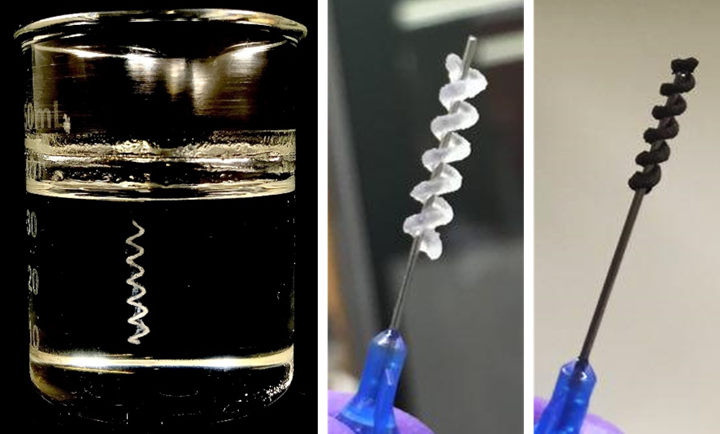
The support bath allows for non-planar 3D printing as illustrated by this spiral. From left to right: The part as printed, still in the thixotropic bath; the crosslinked polymer; the final ceramic part following pyrolization.
Once the 3D print is complete, the entire bath is placed in an oven for the curing process to crosslink the PDC into a solid polymer all at once. The mineral oil-based bath solution was selected in part to enable this. While a water-based bath would boil and evaporate by the time it reached 160°C, mineral oil has a boiling point of about 300°C, enabling the thixotropic bath to not only withstand the curing process but also be reused afterward. The crosslinked intermediary part is then removed from the gel bath and cleaned with ethanol before pyrolyzing in a furnace for several hours at 900°C. The preceramic polymer gives off substances, including H2, H2O and CO2, during this process, resulting in shrinkage of about 15%. After pyrolyzation, the part will have achieved its final geometry and ceramic material properties.
SiOC ceramic parts made with this technique have been shown to withstand temperatures upward of 1,200°C. These parts also have an unintended advantage as a result of the process: Residue left by the support bath means that the final ceramic parts retain a fine layer of silica nanoparticles even after being cleaned and pyrolyzed. The material forms a protective layer against oxidation that might otherwise have to be added in a separate step, Minary says.
Applications for 3D Printed Ceramics
UTD’s experiments into 3D printing SiOC preceramic polymer with a thixotropic bath have been promising so far, but the research into its potential has only just begun. One future avenue of that research might be tweaking print parameters to see how far the geometric capabilities of the process can be pushed. Scaling up prints would be an advantageous way of building large, complex ceramic parts, but the process could also be used to make smaller features as well. Typically, extrusion 3D printing has a resolution only as good as the nozzle diameter, for example, but with the support bath, UTD’s 3D printer can actually achieve resolution finer than this.
“We used a 1-mm-diameter nozzle and you would think that the filament that comes out is 1-mm, but that’s not necessarily true,” Minary explains. “You can take the same nozzle, with the same flow rate, and just increase the speed to get finer resolution. The nozzle area is constant, but the cross-sectional area of the ‘filament’ varies with the speed of the nozzle.”
Tuning the support bath and print speed would also enable the 3D printing of polymer-derived ceramics other than SiOC. “The process is resin-agnostic,” Minary explains. “You can essentially buy any polymer-derived ceramic off the shelf and print with it.” The technique could be applied to materials such as SiBCN, for example, which can withstand temperatures up to 1,700°C for an even wider scope of applications. Another possibility might be 3D printing PDCs reinforced with chopped carbon fiber to help increase the fracture toughness of ceramics, opening them to more structural and load-bearing applications.
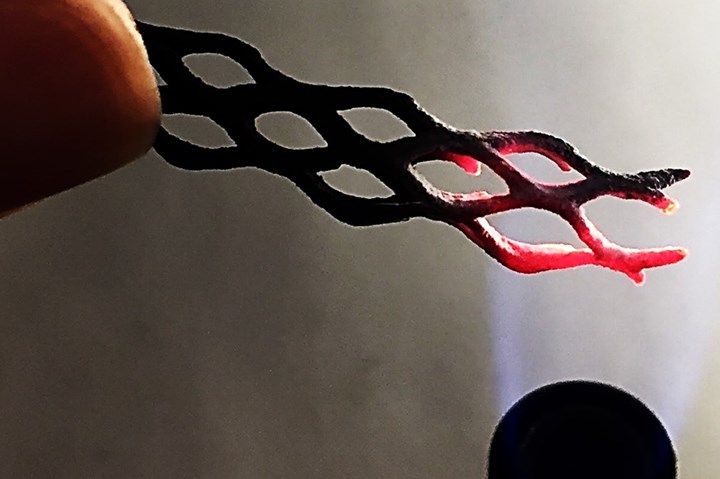
Polymer-derived ceramics can withstand high temperatures without melting or serious deformation. This truss beam structure 3D printed from SiOC is shown after pyrolysis, in its finished ceramic state, over a flame of about 1,400°C.
In the long run, parts requiring high thermal conductivity, corrosion resistance, lightweighting or some combination of all three could benefit from the geometric freedom, affordable feedstock and ease of manufacturing with this process. Capacitors, parts with embedded sensors and more are all possible applications, as are larger structural components, even those that today are made with metal.
“We can scale up this process to create larger structures and broaden the scope of applications,” Minary says, pointing out that this broadening is not only possible but may be necessary for certain industries to advance, whether or not that is clear to those industries today. “Hypersonics, high-temperature gas turbines, high-pressure heat exchangers, even microelectronics — all of them need ceramic additive manufacturing.”
Related Content
Understanding PEKK and PEEK for 3D Printing: The Cool Parts Show Bonus
Both materials offer properties desirable for medical implants, among other applications. In this bonus episode, hear more from Oxford Performance Materials and Curiteva about how these companies are applying PEKK and PEEK, respectively.
Read MoreAircraft Ducts 3D Printed in Composite Instead of Metal: The Cool Parts Show #68
Eaton’s new reinforced PEKK, tailored to aircraft applications, provides a cheaper and faster way to make ducts compared to formed aluminum.
Read MoreWhat Does Additive Manufacturing Readiness Look Like?
The promise of distributed manufacturing is alluring, but to get there AM first needs to master scale production. GKN Additive’s Michigan facility illustrates what the journey might look like.
Read MoreActivArmor Casts and Splints Are Shifting to Point-of-Care 3D Printing
ActivArmor offers individualized, 3D printed casts and splints for various diagnoses. The company is in the process of shifting to point-of-care printing and aims to promote positive healing outcomes and improved hygienics with customized support devices.
Read MoreRead Next
3D Printed Ceramics Serve As Both Bone Graft and Support
Bioceramics including tricalcium phosphate and zirconia have been used to replace and stabilize human bone in reconstructive surgeries. Now, 3D printing brings customization and new design opportunities to these medical devices.
Read MoreSolving a Materials Challenge in Soft Robotics
A team from the University of Minnesota and the Army Research Lab has developed processes for fully 3D printing soft dielectric elastomer actuators without postprocessing steps.
Read MoreVideo: Strength of Aluminum Metal Matrix Composite Versus Aluminum Alloy
The Aluminum MMC demonstrates a yield strength greater than 1018 steel. Additive opens the way to applying advanced materials such as this.
Read More