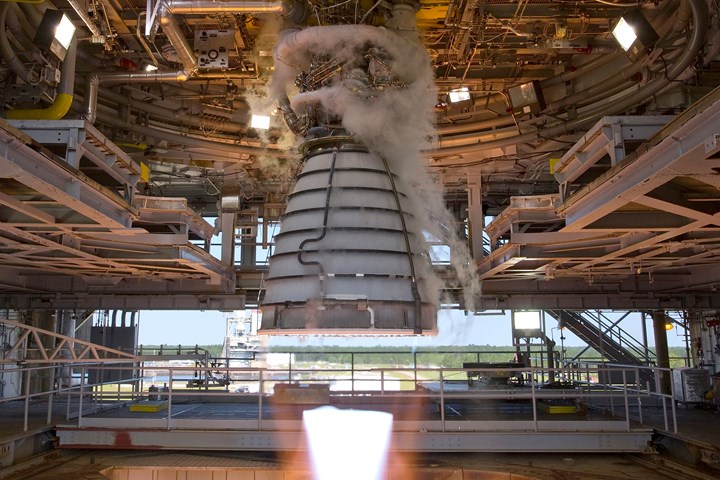
An RS-25 engine hot fire test from inside A-1 test stand at NASA's Stennis Space Center.
Photo Credit: Aerojet Rocketdyne
The Apollo space program that first landed humans on the moon and marked a signature Cold War victory against the Soviet Union has been called “the greatest engineering and exploration feat in human history.” More than 50 years later, NASA is finally closing in on a series of missions that, if fully realized, will exceed the ambition of the original moon landings.
With a current budget hamstrung by federal spending levels that are a fraction of those dedicated to the Apollo program during the 1960s, NASA is turning to private partnerships and advanced manufacturing technologies — including a growing reliance on additive manufacturing (AM) — to make up the deficit. But not all partnerships are created equal, and not all are new.
Aerojet Rocketdyne has been the prime contractor for the high-performance RS-25 engines that once powered NASA’s Shuttle program — engines that are being updated and soon will be used to propel NASA’s newest heavy lift launch vehicle, the Space Launch System (SLS). It is the SLS that, through several planned missions which NASA hopes to begin late in 2021 with the Artemis program, will be tasked with launching invaluable payloads — including humans — into deep space and eventually to the surface of Mars.
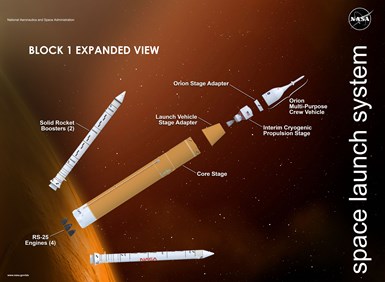
A diagram of NASA’s Space Launch System components, including the four RD-25 engines located at the bottom of the rocket.
Photo Credit: NASA
The RS-25 engines are so critical to the Artemis program that in May of last year, NASA awarded Aerojet Rocketdyne a $1.79 billion contract modification for the production of 18 RS-25 engines to support Artemis and subsequent deep space exploration missions. When added to the existing contract with NASA, which calls for the production of six new RS-25 engines and modifying existing engines from the Space Shuttle program, the value of the total contract of 24 new RS-25 engines is $3.5 billion.
NASA and Aerojet Rocketdyne have already completed hot-fire testing of the new RS-25 engines outfitted with 3D-printed vibration dampening devices known as a pogo accumulator assemblies. On their surface, these “pogos” resemble giant metal balloons. Functionally, they serve a vital role as shock absorbers that dampen oscillations caused by blasts of propellants between the vehicle and the engine. A pogo accumulator assembly stabilizes this so-called “pogo effect,” which makes it critical to astronaut and vehicle safety. In fact, it is this thrust vibration phenomenon that occurred so violently on the Apollo 6 that it shook off panels of the lunar module adapter during flight.

An Aerojet Rocketdyne technician inspects the 3D printed pogo accumulator assembly on an RS-25 development engine at the Aerojet Rocketdyne facility located at NASA's Stennis Space Center. Photo Credit: Aerojet Rocketdyne
The Mission to Mitigate Risk
For more than 30 years, RS-25s were the core engines that powered the Space Shuttle program missions. When that program ended in 2011, there were 16 RS-25 engines left over that are being modified with new controllers — the “brains” of the engine that allow it to communicate with the rocket while managing thrust levels and performing diagnostics. But it is Aerojet Rocketdyne’s latest RS-25 engines that feature parts created via new manufacturing processes, such as a main combustion chamber manufactured via hot isostatic pressing (HIP), and the additively manufactured pogo accumulator assembly.
The pogo assembly consists of two components: the pogo accumulator and pogo-z baffle. Aerojet Rocketdyne has additively manufactured both components via powder bed fusion — a feat that was aided by the company’s 2019 purchase of 3D Material Technologies (3DMT), a Daytona, Florida company that specializes in metal AM for the aerospace, defense, medical and industrial markets. On the pogo accumulator alone, 3D printing eliminated more than 100 welds and reduced costs by nearly 35 percent and production time by more than 80 percent. With each weld comes inspections and possible rework, which means that eliminating those welds can not only make the hardware more reliable, but also smooth the process into a leaner, efficient and cost effective one.
Aerojet Rocketdyne has turned to EOS and Concept Laser machines for the bulk of its metal AM parts, including the pogo, which it prints with Alloy 718 — a high-strength, nickel-based super alloy that can can reliably withstand extreme operating environments, including high pressures and temperature gradients that range from negative 423°F to more than 2,400°F.
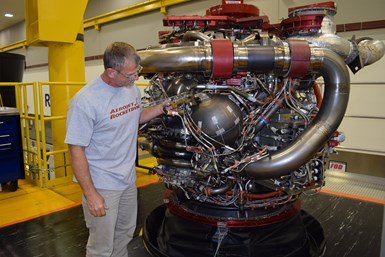
“The pogo accumulator looks like a metal beach ball, but inside is a complex baffling structure to separate the gas and the liquid,” says Rob Brazier, production program manager for Rocketdyne. “It used to include dozens of fillet weld joints that look kind of more like chamfers which were prone to minor indications detectable by NDT (non-destructive testing), causing many hours of rework. But the printed version is beautifully smooth and round, eliminating the detectable flaws in the material. And there's much less stress concentration because it is printed in one fell swoop.”
Andy Hardin is NASA’s engine subsystems manager who oversees the RS-25 engine integration hardware — the lines, ducts, bolts and connecting hardware that pull the engine together. He is also NASA’s additive manufacturing lead at the Marshall Space Flight Center in Huntsville, Alabama. Hardin told me that the pogo was a natural choice for additive manufacturing when it was evaluated by a board at the Marshall Space Flight Center.
“We have a process where basically the designers at Aerojet Rocketdyne are able to bring us what they believed would be good candidates for AM parts,” Hardin says. “They make their case for those parts and we would bring those suggestions to a board at Marshall where we go over the pros and cons of that part being made additively. We have to evaluate the risk, cost savings and several other factors and essentially buy into the part (being 3D printed).”
Hardin says that the decision was made to convert pogo accumulator assembly production to AM even though the 3D-printed version’s design remained almost unchanged. “We didn't really change the function or the form of the part,” Harding says, “although we did add a little bit of structure to change the resonance of that part a little bit to take care of some load issues. Other than that, it's really the same design.”
Though the design has remained stable, even well established production operations have not. The company’s use of additive manufacturing to reduce costs and cut down lead times has been accelerating in recent years, not only with the RS-25 engine, but also with what the company calls “major” metal AM components on a new version of its RL10 engine, the RL10C-X. Robert Lopez, Rocketdyne’s principal machining process engineer, tells me that the influx of new, additively manufactured parts has required new CNC machining processes to achieve the final surface finishes and precise tolerances.
“I wouldn’t call it a challenge necessarily,” Lopez says, “but the methods we use to hold these parts can be very different than with earlier (traditionally manufactured) versions. That’s what has changed the most on the machining side.” Lopez adds that new workholding solutions often come in the form of 3D-printed fixtures, some of which the company prints with nylon polymers as prototypes for the final, machined tool. “There’s a little bit of learning going on here, but we have come up to speed pretty quickly in figuring how we hold these parts,” Lopez says. “These are often contour shapes, and holding them is a little different than holding a fully machined part.”
Lopez also points to the advance of simulation software in recent years — specifically the capabilities of CGTech Vericut software — as a leap forward not only in the ability to finish machine 3D printed parts, but also to optimize cutter tool paths in traditionally manufactured engine components. “If we look at machining as a whole, certainly machine tools are much more accurate. Today it's not so much how deep of a cut we can take and how big of a chip we can throw off the cutter. That's not the game. Now it's, let's get the most efficient cut possible, and that's where we're really seeing gains and efficiency as well as longer cutter life.”
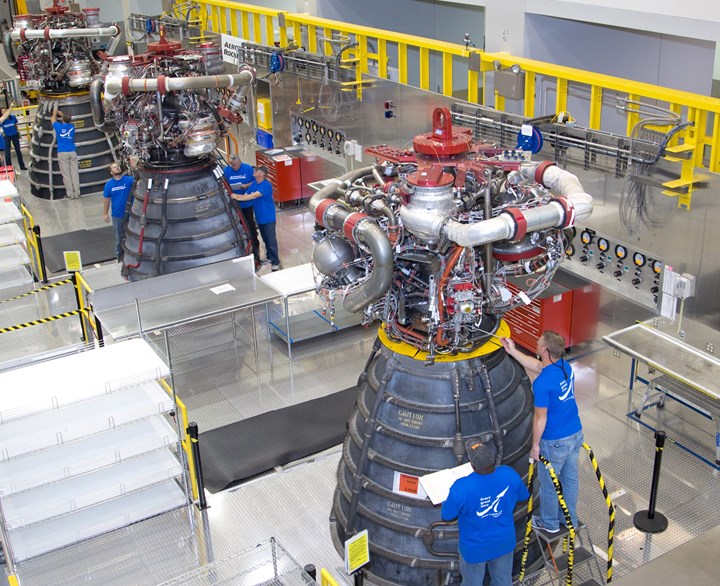
RS-25 engine inspection inside the engine assembly room at Aerojet Rocketdyne's Stennis Space Center Facility. Photo Credit: Aerojet Rocketdyne
Rob Brazier says that these optimized tool paths are vital toward mitigating risk during complex machining processes, both for traditionally machined parts and assemblies that contain 3D-printed parts. “We have to be very careful when cutting long-lead materials,” he says. “To build an RS-25 engine takes a multiple of years, and sometimes you might be two or three years into the cycle before you get to the machining of those components — possibly a critical part — where if there's a mistake made or something doesn't go as we planned, the recovery to that is extremely labor intensive and costly. Sometimes that means forgoing what might be considered an optimum tool path for one that is efficient but maintains a greater reliability, so we don't impact our schedules further down the line.”
Alan Fung, senior engineer and additive manufacturing team leader for Aerojet Rocketdyne, says that one of the greatest benefits of AM ties directly to the development cycle and lead-time risks that the company mitigates through simulation software and careful machining processes. The castings and forging materials take months or longer to acquire, especially when ordered in low quantities. “When we go into casting houses and tell them we want a particular casting, it might be a year before we receive that final casting. And that's really difficult when you're doing development work — when you want to make modifications to geometry and incorporate the design flexibility to optimize the part. But the casting is locked in. And that makes it really hard to take advantage of that design flexibility. But when you get into SLM (selective laser melting), or when you talk about these additive processes, you realize, oh, I can increase this blade angle a little bit. You can do that in development. You can incorporate it and you're not locked into the geometry.”
Four RS-25 engines attached to the core stage for NASA’s Space Launch System rocket for NASA’s Artemis I mission to the Moon.
Photo Credit: Aerojet RocketdyneBuilt for Power
Having launched 135 space shuttle missions, RS-25 engines are considered to be the most tested engines in the world. Of course, for each of the 24 new RS-25s — as well as every other engine NASA will employ for the Space Launch System — it is acceptance testing and certification processes that await at the end of the line for these components.
The first hot-fire tests of an RS-25 rocket engine containing a 3D-printed pogo accumulator — the largest additively manufactured component on the engine — took place in December of 2017. The test was part of what’s been called the SLS Program's RS-25 affordability initiative — a collaboration between NASA and Aerojet Rocketdyne to reduce the engine's overall production costs while maintaining performance, reliability and safety. “This test demonstrates the viability of using additive manufacturing to produce even the most complex components in one of the world's most reliable rocket engines," Eileen Drake, CEO and president of Aerojet Rocketdyne, said at the time. "We expect this technology to dramatically lower the cost of access to space."
That 400-second test took place at NASA's Stennis Space Center, where Aerojet Rocketdyne was able to evaluate the performance of the 3D-printed pogo accumulator assembly. NASA’s Andy Hardin says that the new RS-25s and 3D-printed pogos have been tested beyond maximum load capacity, including a test that purposefully over-pressurized the part until it burst. “We had to make sure we had good analysis about where we predicted it would fail,” he says. “We tested the part to make sure that it behaved that way that we expected. When we hot-fired it, we had strain gauges and accelerometers attached to make sure that we weren't seeing anything that we didn't already expect. And everything worked out great.” Today, the part has undergone more than 5000 seconds of test time. “It has been a great success story for us,” Hardin says.
Much like the FAA can be considered a “certifying partner” as well as the certifying agency for companies such as GE Aviation that produce engines containing 3D-printed parts, NASA is considered Aerojet Rocketdyne’s certifying customer — as well as a partner that collaborates with the company to help streamline manufacturing processes and the ongoing tests that take place at each stage of production.
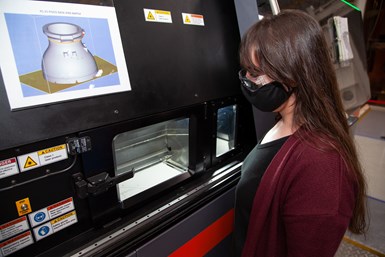
One of the powder bed fusion machines installed at Aerojet Rocketdyne’s headquarters in Los Angeles.
Photo Credit: Aerojet Rocketdyne
“This is a topic that I think a lot of people don’t understand,” Brazier says. “It is a joint effort in which NASA gives us a statement of work required: these are the performance requirements needed at this level of cost. We put together a plan that we think will meet those requirements and partner with NASA to baseline them. There are numerous chief engineer meetings and numerous board meetings needed to come up with criteria to establish how many tests are required and the necessary duration for those tests.” All of this information — especially for parts and components that are being 3D printed for the first time — are baselined and documented in order to establish acceptance testing criteria for newly created similar parts.
Alan Fung, Rocketdyne’s AM team leader, says that the challenge of generating certification requirements for 3D-printed engine components is similar across deep space exploration agencies such as NASA or commercial aviation agencies such as the FAA — namely, you have to to generate those requirements on fly during development. Set requirements and standards didn’t exist. “When you're talking about advanced technology, it's very complicated when someone's making the rules as you're also developing them at the same time,” he says. We have we have people from NASA on site with us, and we have several meetings a calls each week. Oftentimes those calls are simply, ‘We had this thing happen with this part. How is it happening on your side? Are yours okay?’ We just go back and forth.” Fungs says that this collaboration is necessary to make safe parts from advance technologies.
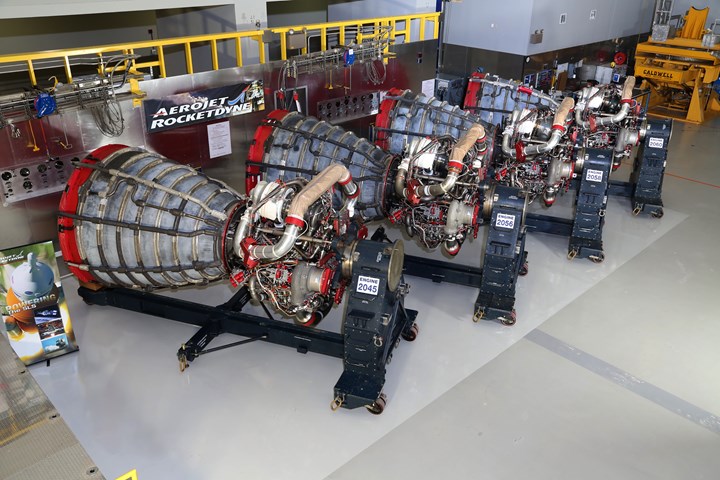
Aerojet Rocketdyne displays the four RS-25 engines slated to fly on Artemis-1, the first flight of NASA's SLS rocket, at its facility located at NASA's Stennis Space Center. Photo Credit: Aerojet Rocketdyne
In October of 2017, NASA published its central certification document for additive manufacturing, called the Marshall Space Flight Center (MSFC) Technical Standard. The goal of this MSFC Technical Standard is to provide “a framework for the implementation of Laser-Powder Bed Fusion Additive Manufacturing parts into spaceflight applications requiring high reliability.” Fung says that achieving these standards requires a functional partnership between NASA and Rocketdyne engineers who are tasked with creating standard, reliable processes in a cost-efficient manner. If experimenting with a new additive process risks wasting thousands of pounds of high-cost metal powder, for example, it may be possible to produce testing data through simulation or other testing methods that will achieve the same reliability.
“That is kind of our way of going back and forth with NASA to help implement realistic requirements while also getting the right controls,” Fung says. “If we have a concern about a part or process, we make recommend a joint study go figure it out. We may conduct that study in parallel or together.” In fact it was that kind of collaboration that helped generate the MSFC Technical Standard, which has been used as a guideline for or several other regulating agencies such as the FAA. “All those regulating bodies talk to each other and build off of what we’re doing.”
The RS-25 engine does have one key advantage when it comes to certifying newly produced components, however. The heritage RS-25 engines that powered the Space Shuttle missions have more than 1.1 million seconds of hot-fire time while successfully powering 135 Space Shuttle missions. Ground test engines have gone in excess of 100 hot fire tests, including overstress conditions and extended durations. Some individual RS-25 engines have been re-flown as many as 21 times. This flight time has provided scores of data and information that can be incorporated into the new versions of the RS-25s, and inform new processes such as additive manufacturing that are being used to increase reliability while decreasing cost and lead times.
“We have a lot of detail, information and data from the heritage engines that we're incorporating,” Brazier says. “And what we're doing now is really looking at those previously existing requirements while building in production and cost efficiencies while maintaining the performance they need to lift this much larger vehicle. It's going to take four RS-25 engines to lift this vehicle to the Moon and beyond. We’re pushing that engine past its former capabilities to make a very reliable, very robust engine that’s built for power.”
Related Content
New Zeda Additive Manufacturing Factory in Ohio Will Serve Medical, Military and Aerospace Production
Site providing laser powder bed fusion as well as machining and other postprocessing will open in late 2023, and will employ over 100. Chief technology officer Greg Morris sees economic and personnel advantages of serving different markets from a single AM facility.
Read More“Mantis” AM System for Spacecraft Uses Induction for Deposition
The metal 3D printing system melts wire without lasers. 30-foot-diameter parts are built on a rotary-feed system that eliminates the need for a large machine frame or gantry.
Read MoreAt General Atomics, Do Unmanned Aerial Systems Reveal the Future of Aircraft Manufacturing?
The maker of the Predator and SkyGuardian remote aircraft can implement additive manufacturing more rapidly and widely than the makers of other types of planes. The role of 3D printing in current and future UAS components hints at how far AM can go to save cost and time in aircraft production and design.
Read More3D Printed Golf Putter Shafts: AM Tailors Weight Distribution for an Optimized Putting Stroke
Snarr3D offers high-performance golf putters with aesthetic features made possible through 3D printing. In manipulating the weight distribution of the golf putter shaft, Snarr3D aims to enhance accuracy and performance to give golfers the equipment suited to their individual game.
Read MoreRead Next
Mission Critical: An AM Breakthrough in Commercial Aviation
The GE9X is the first commercial aircraft engine to reach production with significant additive content. The story of GE’s accomplishment and why it matters.
Read MoreManufacturing in Space
An additive manufacturing process was recently tested in zero-gravity conditions in an effort to develop solutions for manufacturing in outer space. One thing about additive processes—they don’t create loose chips!
Read More3D Printed Polymer EOAT Increases Safety of Cobots
Contract manufacturer Anubis 3D applies polymer 3D printing processes to manufacture cobot tooling that is lightweight, smooth and safer for human interaction.
Read More